Associated Sub-Fields:
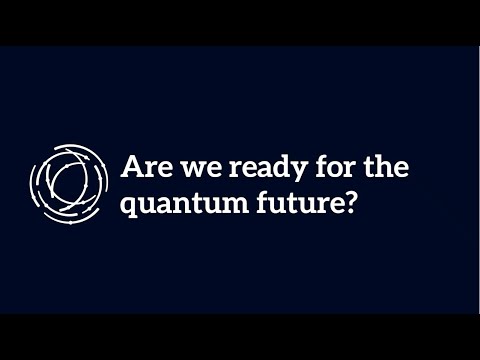
The basic premise of quantum computing is that quantum particles such as atoms, their subatomic components and photons of light (and certain carefully-managed larger objects) can operate in “quantum superposition” states that allow them to encode information in an unprecedented manner. Today’s familiar “classical” machines store and process information encoded as the binary digits (bits) 0 or 1. Quantum bits (or qubits) can not only be states 0 and 1 but can also exist in “superposition states”, where the bit can be both 0 and 1 until observed. Leveraging quantum superposition and quantum entanglement, qubits can perform computational routines that are not possible on classical machines.
Qubits must be isolated from the large-scale classical world, otherwise the sub-atomic quantum properties that give them their unique powers are overwhelmed by classical noise. The result is errors in the computations that are extremely difficult to contain. Nonetheless, progress in isolation, noise mitigation and error correction appears to be accelerating, and quantum computing that outperforms what is reasonable and possible on a classical computer could be achieved within the next five years.5
In order to fulfil all their potential as currently understood, it is likely that quantum computers will need to operate with hundreds of thousands of “physical” qubits (for information processing, these are grouped into structures known as “logical” qubits). However, useful computations are possible with far fewer qubits. This is partly because quantum computers will not simply replace or supersede classical machines, but will provide a novel set of capabilities that can supplement classical capabilities in a hybrid quantum-classical setup.
Applications are diverse, but one extremely promising area is quantum simulation. Because they follow the same rules as atoms and molecules, quantum computers can operate as controllable demonstrators of what is possible within physical materials. This is why they will be so useful for tasks such as innovation in battery technology,6 and drug design for medical intervention.7 Another much-publicised task is decryption. Quantum computers pose a threat to many of today’s cryptographic protocols, whose security relies on mathematical routines that are inaccessible only to classical computation.8 Because the mathematical routines followed by quantum computers are fundamentally different, this security is compromised by the existence of suitably powerful quantum processors that can run a computation known as Shor’s algorithm. This is why a number of governments around the world are now investing heavily in “post-quantum” cryptography.9
KEY TAKEAWAYS
After more than 40 years in development, quantum computing is coming of age, and looks likely to become a multi-billion-dollar industry over the next couple of decades. Though progress has been faster than expected, Quantum hardware development remains a challenge, and there is still no consensus over which of the many hardware approaches will eventually achieve scaleable fault-tolerant quantum computation. Similarly, Quantum algorithms design is still in its early stages, and the number and range of applications that are uniquely suited to quantum computing remain small. Because noise-inducing disturbances of any kind are a fundamental obstacle to quantum computing, a significant effort is being made in the field of Quantum error correction and noise mitigation. Here too, there is reason for optimism, as implementations are growing in complexity. Implementations of Near-term applications of quantum computing are achieving not only proofs of principle, but also rudimentary — and promising — advances in areas such as battery development and cancer treatment.
Anticipatory Impact:
Three fundamental questions guide GESDA’s mission and drive its work: Who are we, as humans? How can we all live together? How can we ensure the well-being of humankind and the sustainable future of our planet? We asked researchers from the field to anticipate what impact future breakthroughs could have on each of these dimensions. This wheel summarises their opinions when considering each of these questions, with a higher score indicating high anticipated impact, and vice versa.
- Anticipated impact on who we are as humans
- Anticipated impact on how we will all live together
- Anticipated impact on the well-being of humankind and sustainable future of our planet
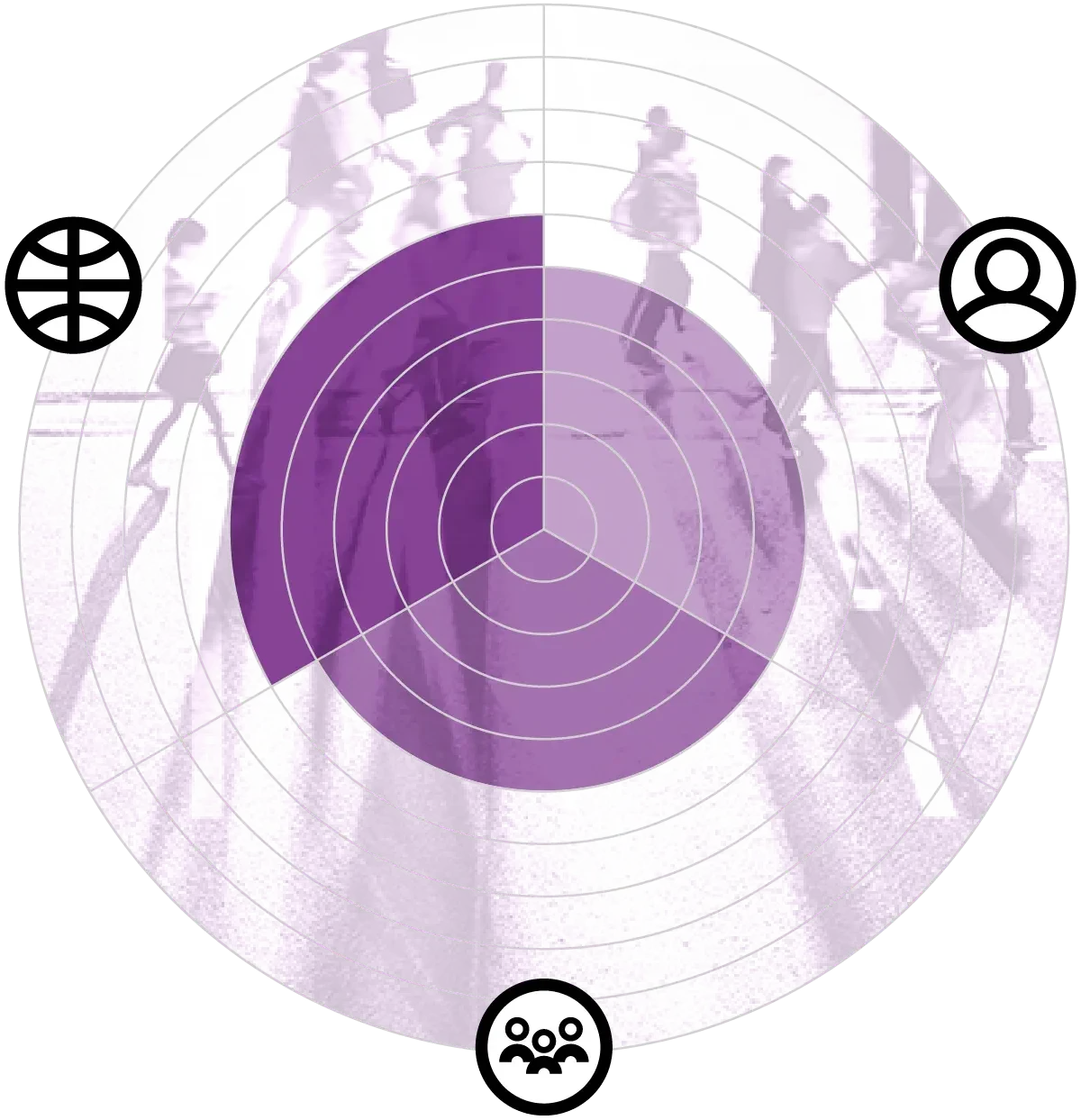