Future Horizons:
10-yearhorizon
Rational design comes of age
25-yearhorizon
Synthetic biology is integrated into other technologies
The most obvious potential is in chemistry and materials science, as micro-organisms such as bacteria or yeast can be engineered to synthesise chemicals22 and materials.23 In effect, living cells are used as factories.24 Thanks to catalytic enzymes, biological systems can often perform syntheses at lower temperatures and pressures than traditional chemical systems, saving energy and reducing greenhouse-gas emissions. There is untapped potential in the engineering of microbial communities and multicellularity, rather than single cells, for these purposes.25
As well as purely biological systems, synthetic biology may be integrated with other forms of technology. For instance, there is growing interest in synthelectronics, which fuses electronics and synthetic biology.26 Living photovoltaic solar cells27 are one possible application.28
An area of increasing interest is the use of synthetic biology to produce food and its ingredients. Examples of this include the use of microbial cells to produce specific molecules such as vitamins, the use of plant cells and biomass to make plant-based meat alternatives, and the use of animal cells to make cultivated meat.29
Finally, many actors are attempting to apply synthetic biology to agriculture. For instance, it may be possible to re-engineer photosynthesis, the process by which green plants use sunlight to make sugars from CO2. More efficient photosynthesis could lead to increased crop yields.30 In the longer term, it may also be possible to engineer the fates of plant cells, optimising the morphology of the plants for new environments.31
Manufacturing, industry and agriculture - Anticipation Scores
The Anticipation Potential of a research field is determined by the capacity for impactful action in the present, considering possible future transformative breakthroughs in a field over a 25-year outlook. A field with a high Anticipation Potential, therefore, combines the potential range of future transformative possibilities engendered by a research area with a wide field of opportunities for action in the present. We asked researchers in the field to anticipate:
- The uncertainty related to future science breakthroughs in the field
- The transformative effect anticipated breakthroughs may have on research and society
- The scope for action in the present in relation to anticipated breakthroughs.
This chart represents a summary of their responses to each of these elements, which when combined, provide the Anticipation Potential for the topic. See methodology for more information.
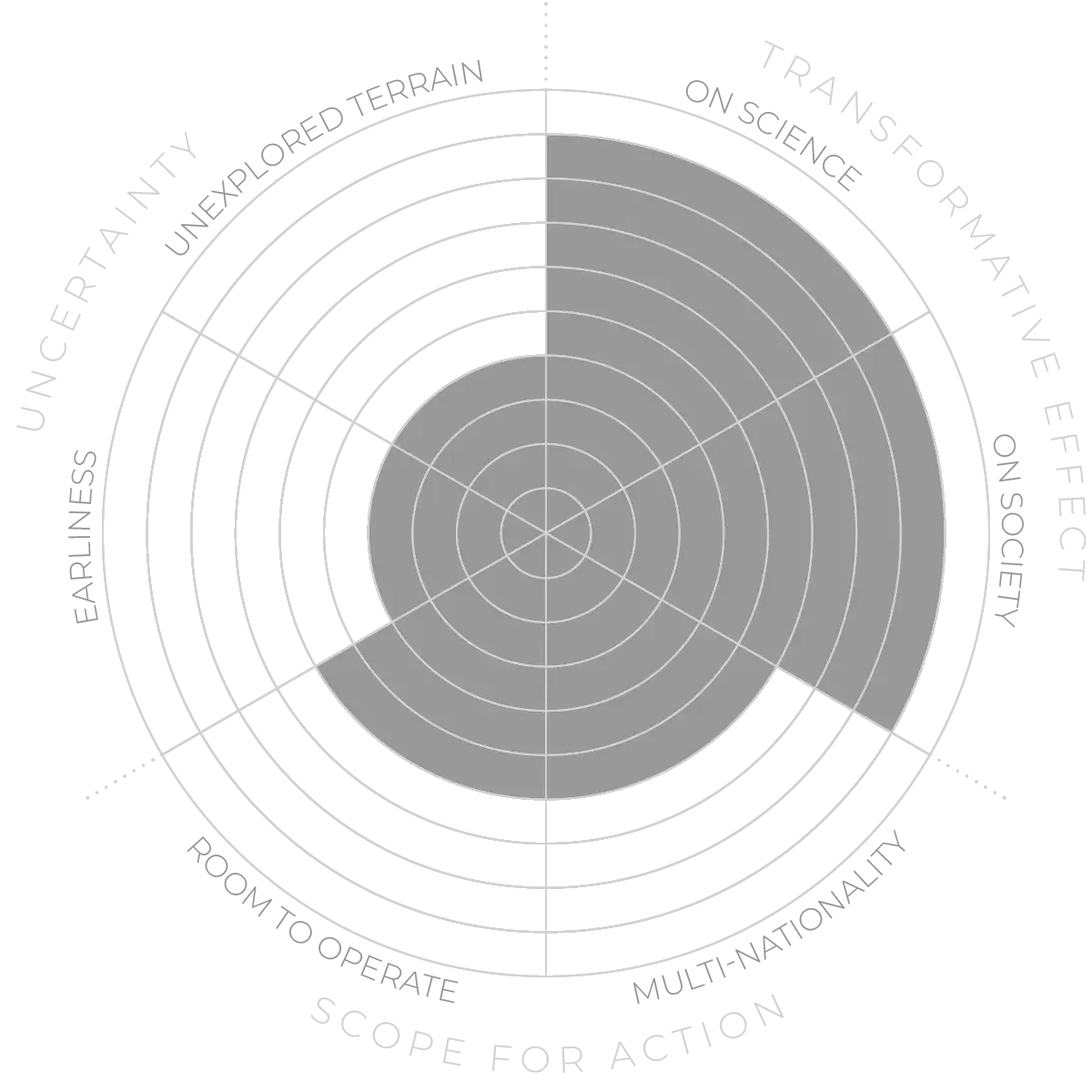